Reading Time – 10 Minutes, Difficulty Level 2/5
Genetic diversity refers to the variety of genetic material within a population, species, or group of organisms. It encompasses the range of different alleles and genetic traits present within individuals of a population and is the result of the combination of different alleles at various gene loci, leading to variations in phenotypic characteristics such as physical appearance, behaviour, and physiological processes.
There are several factors that may contribute to genetic diversity, including sexual reproduction, mutation, recombination, and gene flow – the movement of genes between populations. Genetic diversity holds great importance for the health and adaptability of populations and species, providing the raw material for evolution by natural selection, as well as for organisms to adapt to changing circumstances, resistance to diseases, and environmental challenges.
Maintaining genetic diversity is essential for the long-term survival of populations. Factors such as inbreeding can lead to a lack of genetic diversity and in turn, a reduction of fitness and increased risk of extinction. Contemporary conservation efforts are often focused on preserving or promoting genetic diversity, especially in the case of endangered species, in order to enhance their ability to survive changing environmental conditions. Ecosystem resilience and recovery are shown to be intricately linked to genetic diversity, especially in extreme weather conditions or significant temperature changes.
Genetic Drift
Genetic drift is a mechanism of evolution that occurs when there are random fluctuations in the frequencies of alleles (different forms of a gene) within a population over successive generations. Natural selection is driven by the differential reproductive success of individuals with certain traits whereas genetic drift is a stochastic process. Genetic drift can lead to changes in the genetic makeup of a population, even when the alleles that are involved do not necessarily confer a selective advantage or disadvantage (Kardos et al 2016).
There are two main forms of genetic drift: bottleneck effect and founder effect.
Bottleneck Effect
A bottleneck effect occurs when a population is reduced in size rapidly, often due to an event such as a natural disaster or disease outbreak. The original diversity of the population may not be represented by the surviving individuals, with the subsequent generations inheriting the reduced genetic variation. In larger populations, the effects of genetic drift are generally less significant as the random changes in allele frequencies are diluted by the overall size of the population.
Founder Effect
The founder effect occurs when a new population is established by a small group of individuals, often in an isolated geographical area. The genetic makeup of the founders becomes the basis for the genetic composition of the new population, and rare alleles in the founder group may become more prevalent in the subsequent generations.
Genetic drift is a neutral process and does not necessarily lead to adaptation that would enhance the fitness of a population in its environment. Genetic drift over time can contribute to the divergence of populations, especially when combined with other evolutionary forces such as natural selection, mutation, and migration.
Ne: Effective Population Size
Effective population size (Ne) is a crucial metric reflecting the rate of genetic erosion within a population. It is crucial to understand the link between genetic diversity and effective population size. Ne reflects the size of an idealised population that experiences the same amount of genetic drift as the actual population under consideration. However, populations that are large, do not necessarily ‘act large’ and genetic drift is not, by rule, proportional to census population size (Nc), but is proportional to Ne. When the population is ‘ideal’ in terms of sexually reproducing individuals, Ne = Nc. The criteria of an ‘ideal’ population will include the following four characteristics, in which deviations will decrease Ne:
- There are equal numbers of males and females, all of whom are able to reproduce
- All individuals share equal likelihood to produce offspring, and the number of offspring that each produces varies no more than expected by random chance
- Mating is random
- The number of breeding individuals from one generation to the next is constant.
Any scenario that might increase the variance amongst individuals in reproductive success will, in turn, reduce Ne, the size of an ideal population that experiences genetic drift, at the rate of the population in question (Kliman et al 2008).
A larger Ne generally implies greater genetic diversity, with reduced risk of inbreeding and genetic drift which are mechanisms that can lead to a loss of genetic variation over generations. A larger Ne may aid in the maintenance of gene variants within the population, providing a greater range of genetic material that will contribute to the population’s adaptability to environmental changes, resistance to diseases, and overall evolutionary potential.
Conversely, a smaller Ne can lead to a higher risk of inbreeding, where closely related individuals breed, which may lead to the expression of harmful recessive traits and a decrease in the overall fitness of a population. Genetic drift, which refers to the random changes in allele frequencies over time, also has a more pronounced effect in smaller generations.
Inbreeding
Inbreeding is a phenomenon that occurs when individuals with close genetic relationships, such as relatives, breed with one another, and generally is a consequence of finite populations (where Ne is small, countable and lacks migration) (Kliman et al 2008). Inbreeding and genetic diversity are interconnected when considering their impact on the variation of alleles within a population. Genetic counsellors use the term ‘consanguinity’ for inbreeding, which means ‘of the same blood’. In population genetics, when an individual mates with a relative, the offspring may have two of the same copy of an allele which is ‘identical by descent’ (IBD) from one of the ancestors. The inbreeding coefficient, F, is used to gauge the strength of inbreeding.
F = probability that two alleles in an individual are identical by descent
F is a fixation index that increases in homozygosity as the result of inbreeding.
It is important to note that two alleles that are IBD must be identical by state (IBS). However, two identical alleles may arise without inbreeding in its recent ancestry, implying that IBS does not necessarily imply IBD (Futuyma, 1998).
Reduction in Heterozygosity and Increased Homozygosity
Homozygosity and heterozygosity are terms used to describe the genetic composition of individuals at a specific gene locus. The terms refer to the presence of different alleles (gene variants) at a particular position on a chromosome. Homozygosity refers to where an individual carries two identical alleles at a specific gene locus. It can be classified as either dominant or recessive, where both alleles are the same and represent the dominant form of the gene or the recessive form of the gene. Heterozygosity refers to where an individual carries two different alleles at a specific gene locus, where one allele is dominant, and the other is recessive. The dominant allele typically determines the observable trait.
Known Consequences of Inbreeding
Inbreeding can have several negative consequences, collectively known as inbreeding depression. This can lead to the increased likelihood of individuals inheriting identical alleles from both parents, leading to a reduction in genetic diversity, and an expression of harmful recessive traits. Formally, inbreeding depression is defined by the reduced survival and fertility of offspring born to closely related individuals (Charlesworth and Willis, 2009).
This suggests the presence of genetic variation within a species is found particularly in alleles that have a more direct impact on overall fitness. While inbreeding depression is not exclusive to royal bloodlines, there have been widely documented historical examples from monarchies. Inbred individuals are likely to exhibit reduced overall fitness, lower survival rates, increased susceptibility to diseases, and a higher likelihood of physical and developmental abnormalities. The Habsburg dynasty in Europe has experienced several cases of physical deformities and health issues due to renowned extensive inbreeding (23 and Me, 2019). Another example is haemophilia, an X-linked recessive disorder, which is associated with Queen Victoria’s direct bloodline following her marriage to her first cousin, Prince Albert creating an inbreeding coefficient of F = 6.25% (Stevens, 2005).
It is important to note that whilst royal bloodlines are frequently cited as historical examples, the consequences of inbreeding extend beyond royalty. Inbreeding depression can affect any population that practices close interbreeding over multiple generations, including agricultural populations. Modern conservation efforts aim to minimise inbreeding in endangered species, recognising the potential long-term consequences for the health and survival of populations.
Further Consequences of Inbreeding
Inbreeding leads to a reduction in heterozygosity and an increase in homozygosity. Genetic diversity decreases in turn, with the frequency of homozygosity increased. This exposes deleterious recessive alleles that were previously hidden in heterozygous individuals, leading to the expression of harmful traits, reducing fitness of individuals and potentially compromising the ability of a population to adapt to changing environments.
Accentuating the effects of genetic drift, inbreeding can lead to the fixation of certain alleles and the loss of others. With the increased risk of inbreeding depression, where offspring of closely related individuals exhibit reduced fitness and reproductive success, this may be manifest as lower survival rates, decreased fertility, and other health issues. The longer-term viability of populations may be affected significantly, especially where Ne is small.
In conclusion, genetic diversity is fundamentally a representative measure, reflecting population health and genetics. Effective population size (Ne) is a key determinant in maintaining the balance of this diversity, where a larger Ne fosters resilience to inbreeding and genetic drift. Conversely, inbreeding, often exemplified by a smaller Ne, poses substantial risks through reducing genetic diversity and can lead to inbreeding depression.
Conservation efforts have recognised this vital link between genetic diversity and population diversity with the aim of mitigating the associated risks of finite population sizes and inbreeding, in order to ensure the adaptability and long-term survival of endangered species (Roff, 1997). Comprehension of the dynamics underlying genetic diversity, Ne, and the repercussions of inbreeding are paramount for effective conservation and breeding practices in our ever-changing natural world.
References and Further Reading
23andMe Blog. (2023). How Inbreeding Doomed the House of Habsburg. https://blog.23andme.com/articles/inbreeding-doomed-habsburgndMe Blog
Charlesworth, D., & Willis, J. H. (2009). The genetics of inbreeding depression. Nature reviews. Genetics, 10(11), 783–796. https://doi.org/10.1038/nrg2664
FUTUYMA, DJ 1998. Evolutionary Biology. Chapter 11 (pp. 297-314)
Huisman, J., Kruuk, L. E., Ellis, P. A., & Pemberton, J. M. (2016). Inbreeding depression across the lifespan in a wild mammal population. Proceedings of the National Academy of Sciences, 113(13), 3585-3590. https://doi.org/10.1073/pnas.1518046113
Kardos, M., Taylor, H. R., Ellegren, H., Luikart, G., & Allendorf, F. W. (2016). Genomics advances the study of inbreeding depression in the wild. Evolutionary applications, 9(10), 1205–1218. https://doi.org/10.1111/eva.12414
Kliman, R., Sheehy, B. & Schultz, J. (2008) Genetic Drift and Effective Population Size. Nature Education 1(3):3
Minter M, Nielsen E, Blyth C, Bertola L, Kantar M, Morales H, Orland C, Segelbacher G and Leigh D (2021) What Is Genetic Diversity and Why Does it Matter?. Front. Young Minds. 9:656168. doi: 10.3389/frym.2021.656168
National Institutes of Health (US); Biological Sciences Curriculum Study. NIH Curriculum Supplement Series [Internet]. Bethesda (MD): National Institutes of Health (US); 2007. Understanding Human Genetic Variation. Available from: https://www.ncbi.nlm.nih.gov/books/NBK20363/
Roff, D.A. (1997). Bottlenecks, Finite Populations, and Inbreeding. In: Evolutionary Quantitative Genetics. Springer, Boston, MA. https://doi.org/10.1007/978-1-4615-4080-9_8
Stevens, R. (1999). THE HISTORY OF HAEMOPHILIA IN THE ROYAL FAMILIES OF EUROPE. British Journal of Haematology, 105(1), 25-32. https://doi.org/10.1111/j.1365-2141.1999.01327.x
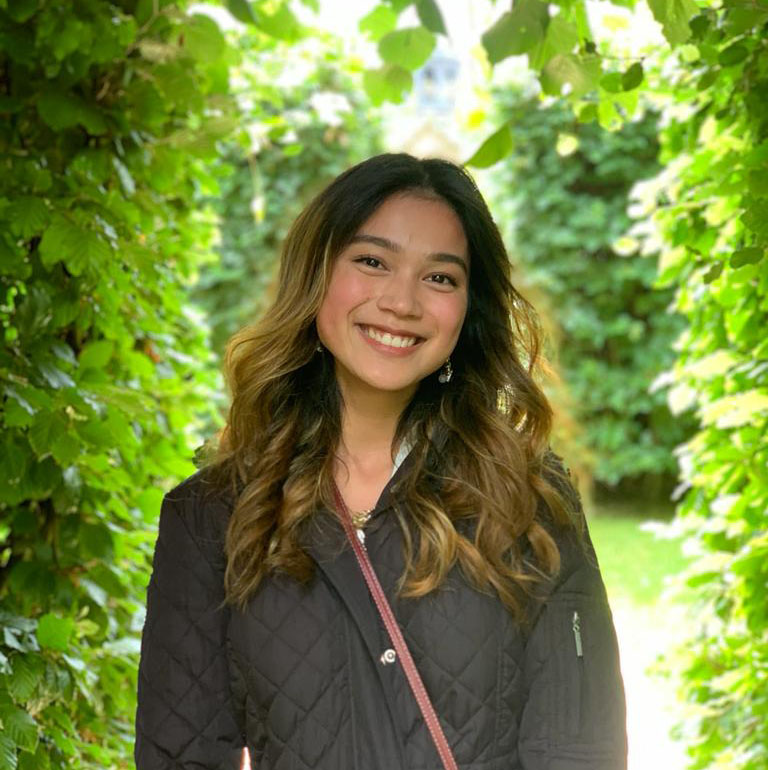
A former professional badminton player turned STEM enthusiast, Basia holds an MSc in Evolutionary Genetics from the University of Edinburgh.
She’s passionate about all things small, from genes to viruses and other microorganisms, and has a particular interest in viral recombination from a phylogenetic and phylodynamic perspective.
1 Comment
A very interesting informative essay providing an understanding of the topic in favour of genetic diversity.