Reading Time – 6 Minutes, Difficulty Level 3/5
From Sunlight to Electricity
Every hour, the earth absorbs over 400 exajoules (that’s 108 joules) of light energy from the sun. For reference, the total global energy production in 2019 was estimated to be 617 exajoules, so it would take less than two hours of sunlight to power the planet for a whole year. The tricky part is capturing all that energy, and converting it into usable electricity.
Most of the electricity we produce comes from the sun indirectly. Plants harness solar energy through photosynthesis, eventually decaying and transforming into coal and natural gas over millions of years. Wind is generated due to temperature variations in the air resulting from uneven solar heating of the planet. Additionally, water evaporates from oceans and lakes, forming clouds that precipitate at higher elevations, ultimately shaping rivers for hydroelectricity.
In order to capture solar energy directly, we can use solar panels.
From Atoms to Semiconductors
So, how do solar panels turn light into flowing electricity?
To understand this, we need to understand atoms.
An atom is made of a heavy, positively-charged nucleus which is surrounded by a cloud of fast-moving, negatively-charged electrons. Quantum mechanics dictates that there is a set of stable paths (called orbitals) that electrons can follow, and only two electrons can occupy the same orbital at a time. Each orbital has an associated energy, so an electron that moves between two orbitals must gain or lose the appropriate amount of energy.
For example, the lowest energy orbital of hydrogen has an energy of -13.6 eV (electron volts) and the second-lowest has an energy of -3.4 eV. Note, these values are negative, because zero energy is defined to be an electron that is free from its orbit around the nucleus. For an electron to drop from the second orbital to the lowest orbital, it must lose 10.2 eV by emitting a photon of light. In this case, the energy corresponds to ultraviolet light.
When many atoms come together to form a solid material, their orbitals combine to form new molecular orbitals. Some are localized to a single atom, and some of which allow electrons to flow throughout the material. The highest energy orbital that is localized is called the valence band and the lowest energy orbital that is delocalized is called the conductance band. The difference in energy between these two states is called the band gap.

Source: Conduction and Valence Band in Semiconductors by Nuclear Power
In conducting materials, like metals, there is no band gap. Electrons in the valence band can easily travel to the conductance band and flow throughout the material. In insulators, like plastics or ceramics, the band gap is huge, trapping electrons in the material and preventing current from flowing. Semiconductors are the perfect median, with a small band gap that electrons can cross if they absorb some energy.
From Instability to Diodes
Once an electron in the valence band absorbs a photon of light, it jumps to the conductance band, but then what? How do we get this electron to move and form current?
To accomplish this, we need to make our semiconductor a bit unstable by replacing some of the atoms in a process called doping. Atoms have to fulfill two conditions to be stable. First, they have to be close to electrically neutral. Second, they have to have a certain number of electrons in their orbit. The reason why is a story for another day, but atoms will generally be stable if they have the same number of electrons as a noble gas.
P-doping occurs when we add atoms that don’t have enough electrons. N-doping occurs when we add atoms with too many electrons. If we attach a piece of each type of semiconductor to each other, electrons will flow from one piece to another, creating a small eclectic field. This structure is called a diode and it is the basis of most modern day electronics.

Source: What is Diode? A Detailed Guide on Diodes by Emma Ashely
From Charge to Current
When sunlight shines onto the diode, electrons absorb the energy and jump to the conductance band. They are then pushed by the electric field toward the n-doped side, leaving behind a positively charged region called an electron hole. An electron will quickly fill the hole, but this just creates another hole, which also quickly gets filled. In this way, the hole “moves” into the p-doped region. Overtime, a charge builds up on either side of the diode.
Instead of letting this charge difference build up, we can attach one end of a conducting wire to each end of the diode. This creates a closed circuit, allowing electrons to freely flow to the positively charged side of the diode, creating a current.
Cost and Optimization
Diodes used in this way are called solar cells. Each solar panel is made up of many solar cells. Currently, the lifetime cost of solar energy is $31 per megawatt hour, cheaper than coal, but still more expensive than wind, natural gas, and nuclear. On the other hand, the cost of solar power is continuing to fall thanks to improvements in efficiency.
For a solar panel to convert 100% of incoming light into electricity, the wavelength of light must have the same energy as the band gap of the semiconductor. The sun produces many wavelengths of light, so this is not the case. Photons with too little energy are not absorbed, and photons with too much energy radiate the excess as heat or longer wavelength light. The maximum theoretical efficiency of a silicon solar panel is 30%.
In practice, modern solar panels vary between 10% and 22% efficiency, with more expensive panels generally performing better. This energy is lost when light reflects off the panel instead of being absorbed, when imperfections in the semiconductor cause electrons and holes to recombine too soon, or when energy is lost as heat in other electrical components. Beyond making silicon-based solar panels more efficient, researchers have been exploring other materials such as perovskites, a class of crystal structures that have achieved efficiencies over 25%.
Conclusion
In summary, solar panels allow us to capture the energy of the sun using the unique electrical properties of semiconductors. Diodes are created by combining an electron-deficient and electron-rich region of semiconducting material.
When it is exposed to sunlight, electrons gain enough energy to move freely about the diode, and a charge builds up. Completing the circuit with a conducting wire creates a direct current that can be used to power electronics. Although other sources of electricity are currently cheaper, solar cell technology is continuing to evolve, bringing about more efficient solar cells each year.
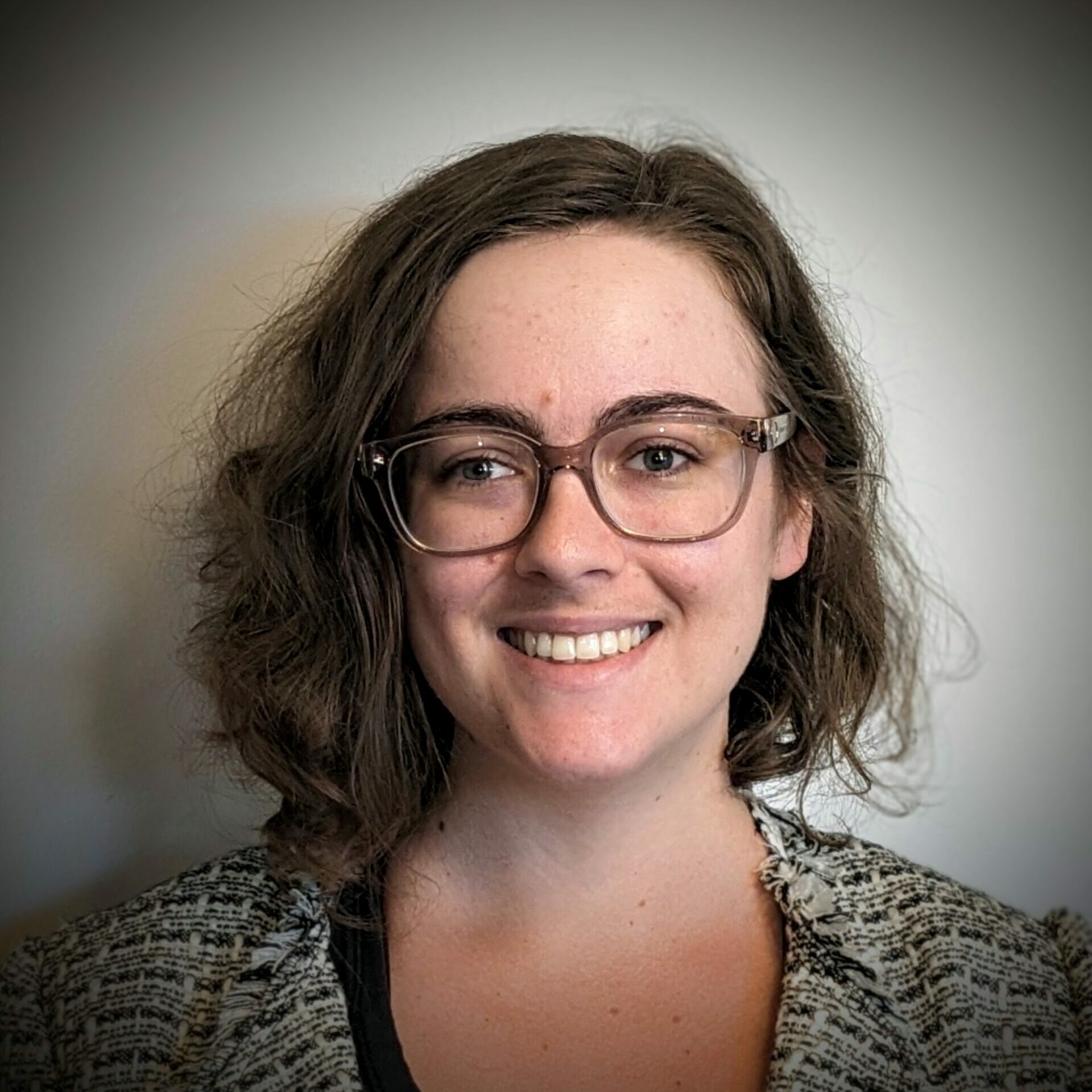
I’m a recent graduate with a B.Sc. in Chemical Physics from the University of Waterloo. I love breaking down how and why things work right down to the atoms! Some of my subjects of interest are quantum mechanics, nanotechnology, and materials science.